Compozit structures Ba0.5Sr0.5Сo1−xFexO3−z, synthesized on the big solar furnace
Abstract
Anion-deficient structures based on the composition Sr0.5Ba0.5Со1−xFexO3−z synthesized from a melt in a solar furnace in a stream of concentrated solar radiation with a density of 100–200 W/cm2 have been studied. Briquettes of the form of tablets based on a stoichiometric mixture of carbonates and oxides of the corresponding metals (SrСО3 + BaСО3 + Со1О3 + Fe2O3) were melted on the focal spot of the big solar furnace. Drops of the melt flowed into the water, cooling at a rate of 103 deg/s. Drops of the melt flowed into the water, cooling at a rate of 103 deg/s. The castings were crushed to a fineness of 63 µm, dried at 400 °C, molded into tablets (samples) (20 mm in diameter and 10 mm high). Samples of the material were sintered in the temperature range 1050 °C–1250 °C. The structure, water absorption and degradation in a carbon dioxide medium were studied on the samples. The crystal lattice of the material had a perovskite structure with a unit cell parameter a = 4.04 Å. The material samples showed increasing water absorption with increasing sintering temperature. There is also a dependence of the resistance of the material structure to the effects of carbon dioxide and water vapor on the sintering temperature. The observed values of structural parameters indicate that the material based on the perovskite Sr0.5Ba0.5Со1−xFexO3−z structures can be used as a catalyst in the production of hydrogen and synthesis gas by reforming and methane oxidation. Preliminary experiments on obtaining synthesis gas showed that the perovskite structures of the composition are not inferior to phosphogypsum in terms of efficiency. However, the implementation of such approaches requires the development and creation of special equipment that makes it possible to control the flows of gases and water into the reaction chamber irradiated by a concentrated flux of high density solar radiation.
Keywords
Full Text:
PDFReferences
1. Galasso FS. Structure, Properties and Preparation of Perovskite-Type Compounds. Pergamon Press; 1968.
2. Goodenough JB. Electronic and ionic transport properties and other physical aspects of perovskites. Reports on Progress in Physics 2004; 67(11): 1915–1993. doi: 10.1088/0034-4885/67/11/R01
3. Peña MA, Fierro JLG. Chemical structures and performances of perovskite oxides. Chemical Reviews 2001; 7(101): 1981–2017. doi: 10.1021/cr980129f
4. Yang JB, Kim J, Woo YS, et al. Magnetoresistance in double perovskites Ba2−xLaxFeMoO6. Journal of Magnetism and Magnetic Materials 2007; 310(2): e664–e665. doi: 10.1016/j.jmmm.2006.10.916
5. Burns G, Dacol FH. Glassy polarization behavior in ferroelectric compounds Pb(Mg{1}/{3}Nb{2}/{3})O3 and Pb(Zn{1}/{3}Nb{2}/{3})O3.Solid State Communications 1983; 48(10): 853–856. doi: 10.1016/0038-1098(83)90132-1
6. Kharton VV, Patrakeev MV, Waerenborgh JC, et al. Methane oxidation over perovskite-related ferrites: Effects of oxygen nonstoichiometry. Solid State Sciences 2005; 7(11): 1344–1352. doi: 10.1016/j.solidstatesciences.2005.08.004
7. Sharma S, Tomar M, Kumar A, et al. Photovoltaic effect in BiFeO3/BaTiO3 multilayer structure fabricated by chemical solution deposition technique. Journal of Physics and Chemistry 2016; 93: 63–67. doi: 10.1016/j.jpcs.2016.02.010
8. Zhang J, Gao X, Deng Y, et al. Comparison of life cycle environmental impacts of different perovskite solar cell systems. Solar Energy Materials and Solar Cells 2017; 166: 9–17. doi: 10.1016/j.solmat.2017.03.008
9. Vassilakopoulou A, Papadatos D, Koutselas I. Light emitting diodes based on blends of quasi-2D lead halide perovskites stabilized within mesoporous silica matrix. Microporous and Mesoporous Materials 2017; 249: 165–175. doi: 10.1016/j.micromeso.2017.05.001
10. Arutyunov VS. Oxidative Conversion of Natural Gas (Turkish). Krasand; 2011.
11. da Rosa AV, Ordóñez JC. Fundamentals of Renewable Energy Processes, 4th ed. Elsevier; 2022. pp. 419–470.
12. Bouwmeester HJM, Burggraf AJ. Dence ceramic membranes for oxygen separation. In: Gellings PJ, Bouwmeester HJM (editors). Handbook of Solid State Electrochemistry, 1st ed. CRC Press; 1997. pp. 481–553.
13. Tang M, Xu L, Fan M. Progress in oxygen carrier development of methane-based chemical-looping reforming: A review. Applied Energy 2015; 151: 143–156. doi: 10.1016/j.apenergy.2015.04.017
14. Teraoka Y, Zhang H, Furukawa S, Yamazoe N. Oxygen permeation through perovskite-type oxides. Chemistry Letters 1985; 14(11): 1743–1746. doi: 10.1246/cl.1985.1743
15. Shao Z, Yang W, Cong Y, et al. Investigation of the permeation behavior and stability of a Ba0.5Sr0.5Co0.8Fe0.2O3−δ oxygen membrane. Journal of Membrane Science 2000; 172(1–2): 177–188. doi: 10.1016/S0376-7388(00)00337-9
16. Chang X, Zhang C, He Y, et al. A comparative study of the performance of symmetric and asymmetric mixed-conducting membranes. Chinese Journal of Chemical Engineering 2009; 17(4): 562–570. doi: 10.1016/S1004-9541(08)60245-1
17. Payzullakhanov MS, Parpiev OR, Avezova NR, Shermatov Z. Hydrogen storage in porous ceramic materials of aluminosilicate composition. Applied Solar Energy 2022; 58(5): 722–724. doi: 10.3103/S0003701X22601338
18. Allaev KR, Avezova NR. Hydrogen—The future of power engineering for the world and Uzbekistan. Applied Solar Energy 2021; 57: 575–583. doi: 10.3103/S0003701X21060025
19. Zeng Q, Zuo Y, Fan C, Chen C. CO2-tolerant oxygen separation membranes targeting CO2 capture application. Journal of Membrane Science 2009; 335(1–2): 140–144. doi: 10.1016/j.memsci.2009.03.012
20. Sunarso J, Hashim SS, Zhu N, Zhou W. Perovskite oxides applications in high temperature oxygen separation, solid oxide fuel cell and membrane reactor: A review. Progress in Energy and Combustion Science 2017; 61: 57–77. doi: 10.1016/j.pecs.2017.03.003
21. Pan Q, Ma L, Du W, et al. Hydrogen-enriched syngas production by lignite chemical looping gasification with composite oxygen carriers of phosphogypsum and steel slag. Energy 2022; 241: 122927. doi: 10.1016/j.energy.2021.122927
22. Paizullakhanov MS, Parpiev OR, Yu RY, Suvanova SL. Features of the extraction of metals from waste in a solar furnace. Applied Solar Energy 2022; 58(3): 433–437. doi: 10.3103/S0003701X2203015X
23. Akhatova JS, Ahmadova KS. Extraction of hydrogen from water using CeO2 in a solar reactor using a concentrated flux of solar radiation. Applied Solar Energy 2022; 58(6): 889–894. doi: 10.3103/S0003701X22060032
24. Paizullakhanov MS, Shermatov ZZ, Nodirmatov EZ, et al. Synthesis of materials by concentrated solar radiation. High Temperature Material Processes 2021; 25(2): 17–29. doi: 10.1615/HighTempMatProc.2021038543
DOI: https://doi.org/10.59400/esc.v1i1.138
(163 Abstract Views, 147 PDF Downloads)
Refbacks
- There are currently no refbacks.
Copyright (c) 2023 M. S. Payzullakhanov, F. Ernazarov, O. Rajamatov, N. Karshieva, A. Holmatov
License URL: http://creativecommons.org/licenses/by/4.0/
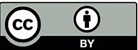
This site is licensed under a Creative Commons Attribution 4.0 International License.